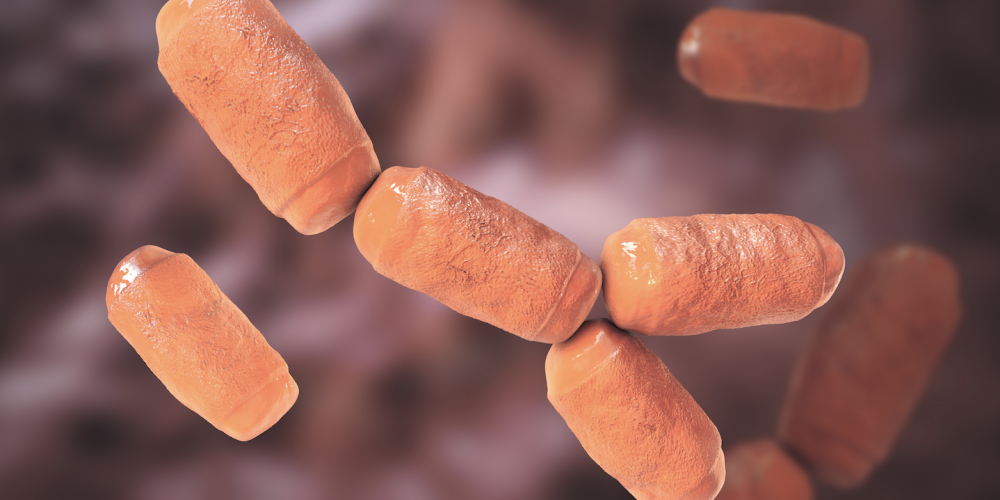
Archaea, representing a group of primordial microbes which were recently found to be very related to humans, often grow best in extreme environments. While many bacteria (which are standing very far from us from a evolutionary point of view) can withstand only small fluctuations in temperature and acidity, Archaea are true survival champions.
PhD research at VUB has discovered the mechanism that underlies this resilience. Archaea, like every other organism, produce heat shock proteins, which ensure that the other proteins in the cells, when distorted by extreme temperatures, are refolded back into their correct form, thereby allowing the cell to survive heat shock. Research has now shed light on how these Archaea âcontrolâ their heat shock proteins and make efficient use of them.
âFor my PhD research, I studied how these microbes deal with temperature stress,â says Rani Baes, a researcher in the research group of Microbiology at VUBâs Faculty of Bioengineering Sciences. âTo do this, I chose the thermophilic model organism of the Crenarchaea: Sulfolobus acidocaldarius. This micro-organism was discovered in the 1970s in a hot water spring and grows optimally at 75°C in an acidic environment with a pH level of around 2-3, which is comparable to almost-boiling lemon juice. I investigated how it reacted to a rapid heat shock, a sudden increase in temperature by 10°C, because the cell has to immediately respond to prevent that cellular molecules (such as DNA, RNA and proteins) will âmeltâ, causing the cell to die.â
Baes discovered that the primary defence mechanism of the model organism is the regulated action of heat shock proteins, which can themselves withstand heat shock and refold other proteins in the cell back to their original functional state. âIn the lab, I simulated the temperature variations that occur naturally in hot springs. My research demonstrated that the defence mechanism of S. acidocaldarius is triggered by a temperature increase of about 10°C. Upon an increase in temperature by 15°C, the cells immediately die. It is therefore important to select the right temperature to investigate how the cells protect themselves.â
To further study the response to heat shock, Baes purified and sequenced the RNA and protein content of the cell. âAt the level of the RNA, we saw a significant response immediately after heat shock,â she explains. RNA is the step between the genetic code in the DNA and the functional protein. âIn bacteria and eukaryotes, the conversion of DNA to RNA upon heat shock is tightly coordinated by a specialised protein. However, our results demonstrated that this is not the case for S. acidocaldarius. Instead, the microbe uses an ancient and less evolved mechanism to regulate the process of transcription. Furthermore, the Archaea cell appears to make a âshort-cutâ in the production process of proteins and by quickly producing heat shock proteins, it can drastically increase the chance of surviving heat shock.â
The fact that S. acidocaldarius employs different regulatory mechanisms makes that it is well adapted to the stressful habitat it lives in. According to Baes,it gives us a deeper insight into how the very first life forms on Earth were able to do the same. âLots of Archaea are extremophiles, organisms that grow optimally in extreme environments,â she explains. âThese hostile environments are reminiscent of how the Earth looked like when life first emerged.Similar environmental parameters can be found today in deep-sea hydrothermal vents on the seabed or in volcanic areas on land, such as geysers or hot springs. About 3.5 billion years ago, when life emerged, such habitats were found all over the Earthâs surface.â
Archaea are one of the three major domains of life and are closely related to humans. The branch of life that evolved into animals, plants and fungi (Eukarya or Eukaryota; these all have a cell nucleus) evolved from such a microbe. In other words, Archaea are the ancestor of Eukaryota.
âUnfortunately, little research is done on archaea,â says Baes. âThat is striking, given that from an evolutionary perspective, it is interesting to understand how these microbes can deal with temperature stress and how these temperature variations are sensed. This can give us insights into how the origin of life on Earth was possible.â